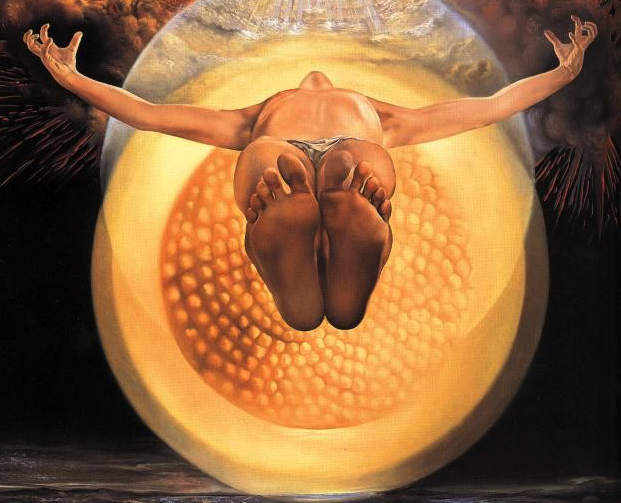
ultrafast endocytosis
The brain is a massively complex network. Nerve cells are linked by releasing and detecting very small packets (called synaptic vesicles) of chemical compounds (called neurotransmitters). The process of releasing and detecting neurotransmitter is called synaptic transmission. The 2013 Nobel prize in Physiology and Medicine was awarded to three researches who identified the molecular details of how cells release these packets at their surfaces.
While that work has clarified a major aspect of neurotransmission, another aspect has remained largely uncharacterized. Every time a synaptic vesicle fuses with the cell surface to release neurotransmitter, the internal stock of packets is depleted. Based on the number of vesicles in a cell and the number of vesicles released while a neuron is active, most neurons would completely deplete their internal stocks in just a few seconds of activity if they didn't recycle the packets from their surface. It is currently unknown how these vesicles are recycled.
One idea that is popular in the field is that neurons recycle the vesicles using the same mechanism that other cells use to bring in nutrients from the bloodstream. The problem with this idea is that the measured speed of this process is much too slow to counterbalance the high rates of release in neurons. A competing idea is that vesicles never fully fuse with the cell surface, but instead just touch the surface long enough to dump their contents before undocking and retreating back into the cell. The problem with this idea is that there is scant evidence of incomplete fusion at the cell surface.
Resolving this issue is no simple task. First, the release sites on the neuron (the synapse) is less than a micron across- about the size of many bacteria, while the vesicles are 30 times smaller, at 30 nanometers across. That's a neat way to pack approximately one trillion synapses into the human brain, but makes it hard to see what's happening. The very best light microscopes that can be made can only see objects 200 nanometers across, so it is literally impossible to see what is happening inside a synapse. The second problem is that a neuron fires in about 10 milliseconds, a quarter of the time between frames of a standard movie film. Finally, neurons usually fire at unpredictable times, so that if you take a picture of a plate of neurons, it is impossible to know for sure what step in the process any neuron was in.
Dr. Watanabe and coworkers have tackled these problems by developing an all new way to look at synapses. To see the inner workings of the synapse, they used an electron microscope, which is able to see things as small as 1 nanometer- 200 times better than any light microscope, and good enough to actually see vesicles in a synapse. To stop the motion of vesicles, they used a new technology called a high-pressure freezer. This device freezes samples into solid ice in 8 milliseconds. A few milliseconds before freezing, the samples are pressurized to 2,100 atmospheres to lock all of the water in the sample in place at the molecular level, so that the frozen tissue is preserved intact and in the exact state it was in when the freezing happened. Finally, they combined these two technologies with a third genetic technique to synchronize the firing of the neurons with the freezing of the sample. They introduced into the neurons a light sensor molecule from a green algae. This molecule is used by the algae to sense and respond to sunlight, but when put into mouse neurons it causes them to fire when a bright light is flashed on them. Putting all of this together, the team used a computer to flash a light, pressurize the sample and then freeze it all in under 20 milliseconds. By flashed the light a few milliseconds earlier on different nerve cells, the team could then make a flip-book of how the cells respond in the fist 200 milliseconds after they fire.
What they discovered has changed how the field understands the synapse. After the nerve cell fires, the vesicles completely fuse with the cell surface and disappear in less than 30 milliseconds, but in 100 milliseconds the cell has taken in bubbles of cell membrane and recycled them into new vesicles. This speed of recycling is 400 times faster than mechanisms that have been seen before. Further experiments by the group demonstrated that ultrafast recycling requires some of the same molecules that the cell uses in slower recycling processes, but they are still working out just how it operates so much faster.